Microbe Chain
The Quadram Institute is looking to reduce the problems caused by microbes in the food chain by delivering an enhanced understanding of the ecology, evolution and survival strategies of pathogens in the food chain, including the drivers of antimicrobial resistance, to improve human health.
Uploaded Use the arrow keys or WASD to control the microbe. Try to keep it inside the circle. The chain behind you slowly grows, making you. ADVERTISEMENTS: The electron transport chains of bacteria (prokaryotes) operate in plasma membrane (mitochondria are absent in prokaryotes). Some bacterial electron transport chains resemble the mitochondrial electron transport chain. Paracoccus denitrificans is a gram-negative, facultative anaerobic soil bacterium. It is a model prokaryote for studies of respiration. When this bacterium grows.
Microbes in the food chain represent a major global challenge to health and the economy, through foodborne infections and through their contribution to the problem of antimicrobial resistance.
Standard servo - TowerPro SG-5010. Standard Size - High Torque - Metal Gear Servo. True Servo Conversion for M.A.C.-R.A.C. “Sort-of-Servos” True servos read a control signal and move a motor or mechanical part as commanded. Some feedback is required to complete the control loop. The standard MAC-RAC runs a motor with two pushbuttons and uses their LED bar graph and the pilot’s sense of stick forces as feedback. This kit includes everything above plus an extra servo, Y-cable, and hardware. The Control Board Only option is available if you want to provide your own servo and hardware. The MRServo-1 can be converted into an MRServo-2 or MRServo-3 using the DIY Conversion Kits. These kits provide the terminal blocks and relay(s) needed for the conversion. True servo conversion for m.a.c.r.a.c. sort.
Working in collaboration with other BBSRC-supported institutes, we are harnessing the latest genomic technologies to track the emergence, evolution and spread of foodborne pathogens in the food chain.
Our research focusses on E. coli, Salmonella, Listeria and Campylobacter, as the major causes of foodborne illness.
Our approach is to gather isolates from across the whole of the food chain, from soil, water, farms, animals, processing factories and humans, to get the fullest possible picture of the genomic epidemiology of these bacteria. Whilst many human isolates have been catalogued, comparatively few have been taken from the food chain environment, so completing this dataset will allow us to identify where foodborne pathogens arise from, and which are the major locations for bacterial communities that potentially could impact health.
Comparative genomics will allow us to identify what drives the emergence of antimicrobial resistance as well as other survival strategies, such as the formation of biofilms that resist cleaning, Salmonella’s ability to colonise plants and animals, and E. coli’s evasion of host defences.
Microbe Chain
The interdisciplinary team of researchers within the Quadram Institute is at the cutting edge of microbial cell biology, genomics, metagenomics, modelling and bioinformatics. Working strategically with partners on the Norwich Research Park and elsewhere, we will be developing novel approaches to sequencing and genomic analysis to better understand the microbiology of the food chain. This will allow us to identify new intervention strategies to stop pathogen spread in the food chain, either working through our ongoing collaborations with food production companies, or through novel techniques, potentially developed through bioprospecting for useful enzymes or probiotic bacterial strains.
Alison Mather
Epidemiology, genomics and antimicrobial resistance of bacteria
John Wain
Bacterial diversity and tropical infections
Justin O’Grady
Rapid infectious disease and foodborne pathogen diagnosis/detection Roland metaza mpx80 driver for macbook pro.
Mark Webber
Investigating the evolution of antimicrobial resistance
FOLIUM Science and Quadram Institute join forces in the fight against anti-microbial resistance
Researchers provide new insights into how antibiotic resistance develops
ViewGenomics helps understand COVID-19 in care homes
ViewCLIMB Receives Honours in 2020 HPCwire Readers’ and Editors’ Choice Awards
ViewA bacterial virus helped the spread of a new Salmonella strain
ViewThe Quadram Institute at the Norwich Science Festival
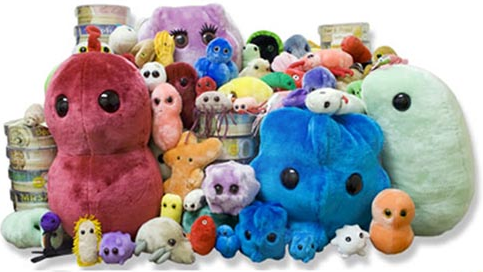
Bacteria Chains

Whole genome sequencing benefits for surveillance of bacteria behind gastroenteritis
Viewby Molly Sargen
figures by Molly Sargen and Nicholas Lue
Microbes (also known as microorganisms) are everywhere: on surfaces we touch, in the air we breathe, and even inside us. As suggested by the name, all microbes are too small to be seen without a microscope. Beyond size, microbes are incredibly diverse. Microbes include bacteria, fungi, and protists. To be able to live harmoniously with all of these microbes, we implement numerous practices to control their growth.
On one hand, there are many harmful microbes we try to avoid. Consider the pathogenic bacteria Listeria monocytogenesand Salmonella entericathatcause foodborne illnesses. We use refrigeration to slow the growth of microbes like these and keep food safe to eat. On the other hand, some microbes perform useful functions and we intentionally cultivate them. For example, bakers use warm temperatures to promote the growth of the yeast Saccharomyces cerevisiaeto make bread dough rise. Some microbes can be both harmful or beneficial in different conditions. For instance, Escherichia coli can cause gastrointestinal illness when ingested but can produce life-saving synthetic insulin in an industrial setting. Understanding the fascinating growth of microbes helps us develop practices to maintain balanced interactions with these microorganisms.
Features of microbes
Microbe Food Chain
Microbes are diverse creatures. Many have unique features and capabilities, yet they share a few common characteristics (Figure 2). Most microbes are made of only one or a few cells. Every microbial cell is surrounded by a cell membrane. The membrane controls the movement of material in and out of the cell. This allows the cell to bring in important material, like nutrients, while expelling waste. Some microbes are also surrounded by a cell wall. The wall provides a structure to enclose the internal components of the cell. Within its interior, each cell carries the DNA encoding its genome. Other structures in the cell perform metabolic functions that are essential for life.
Mechanisms of microbial growth
Microbial growth refers to an increase in number of cells rather than an increase in cell size. Many microbes (including Escherichia coli, Salmonella enterica, and Listeria monocytogenes) are unicellular, meaning they are made of only one cell. The size of any unicellular microbe is limited by the capacity for the essential components of the cell to support its survival. For example, the integrity of the cell wall is impaired when cells become too large. The solution to growing despite limits on cell size is for cells to divide or produce new cells from the original cell. Therefore, the population grows although the size of the individual members of the population remains stable.
Most commonly, a single-cellular microbe divides into two identical new cells during one growth cycle (Figure 3a). The original cell, called the parent cell, makes a copy of its DNA and generates enough material to build the membrane, wall, and molecular machines for two cells. The parent cell slightly increases in size to accommodate these additional materials. Then, the parent cell begins to contract at the middle and a new piece of cell wall is assembled at the site of contraction. This process continues until the parent cell is split into two cells with complete cell walls. The resulting cells are called the daughter cells. Because both daughter cells are identical, cell division is also called replication. Replication in this manner leads to a rapid increase in the number of cells as each daughter cell begins the cycle again by acting as a parent cell. Cell division can look slightly different for microbes with different shapes (Figure 3b), but the key principles remain the same.

As long as the conditions are favorable, one cell produces two new cells in a continuous cycle. Every cycle doubles the number of cells in the population. This is known as exponential growth. Depending on the conditions, the division cycle of E. coli can be as short as 20 minutes. This rapid division leads to a rapid increase in the population size (Figure 3c). Eventually, the population is large enough to have an impact we can detect, such as formation of a physical structure. In a research lab, this might be a “colony,” a mound of bacteria on solid growth media. You might notice this as plaque on your teeth. It takes over a million bacteria to form a visible structure, but this can occur in only about eight hours when conditions are optimal for E. coli.
Some microbes produce new cells asymmetrically. In this situation, one parent cell produces a single daughter cell by a process called budding. During budding the parent cell develops a small protrusion known as the bud. The materials necessary to support a new cell are sent into the bud, which eventually splits from the parent cell to form a new daughter cell. The parent cell continues to make buds, but the budded daughter cells do not divide. Buds can remain connected in a chain or separate into individual cells (Figure 4). Saccharomyces cerevisiae, a yeast used to make bread, is a budding yeast.
There are also multicellular microbes, like algae and the fungi that form molds. For these microbes, multiple cells work together to keep the organism alive. Each cell might perform slightly different functions for the organism. When a parent cell divides, each daughter cell begins to perform specific functions based on its surroundings. The entire organism grows as new cells form and take on new functions. This is similar to the way larger organisms, like animals, grow.
Bacteria Chain Of Infection
Factors affecting microbial growth

All types of microbial growth are heavily impacted by environmental conditions. One of the most critical factors for microbial growth is the availability of nutrients and energy. Microbes need carbohydrates, fats, proteins, metals, and vitamins to survive, just like animals. The process of using nutrients and converting them into cellular material requires energy. Every microbe has unique nutritional requirements depending on the types of molecules it is capable of making for itself. Most microbes are fairly robust, meaning they can find a way to grow in a variety of nutritional conditions. Nonetheless, microbes grow more slowly when nutrients are limited.
Temperature also impacts microbial growth. Most microbes grow optimally within a certain temperature range dictated by the ability of proteins within the cell to function. In general, at low temperatures, microbes grow slower. At higher temperatures, microbes grow more quickly. For instance, pathogens often grow best at normal body temperature, but slowly at cooler temperatures outside the body or when body temperature increases during a fever. Extremely high temperatures usually denature the components required for the cells to survive and are lethal for many microbes. Nonetheless, a few exceptional microbes actually prefer to grow at very high temperatures or very low temperatures. These microbes, known as extremophiles, can grow near hydrothermal vents where the temperature is above boiling or surrounded by solid ice.
Even when nutrients are available and the temperature is right, many other environmental factors can influence the growth of microbes. These include acidity, availability of water, and atmospheric pressure. Each microbe prefers a range of properties for multiple features of the environment. Overall, microbes typically grow best at a specific set of conditions and less well at other conditions (Figure 5). Specific preferences for growth are as diverse as the types of microbes.
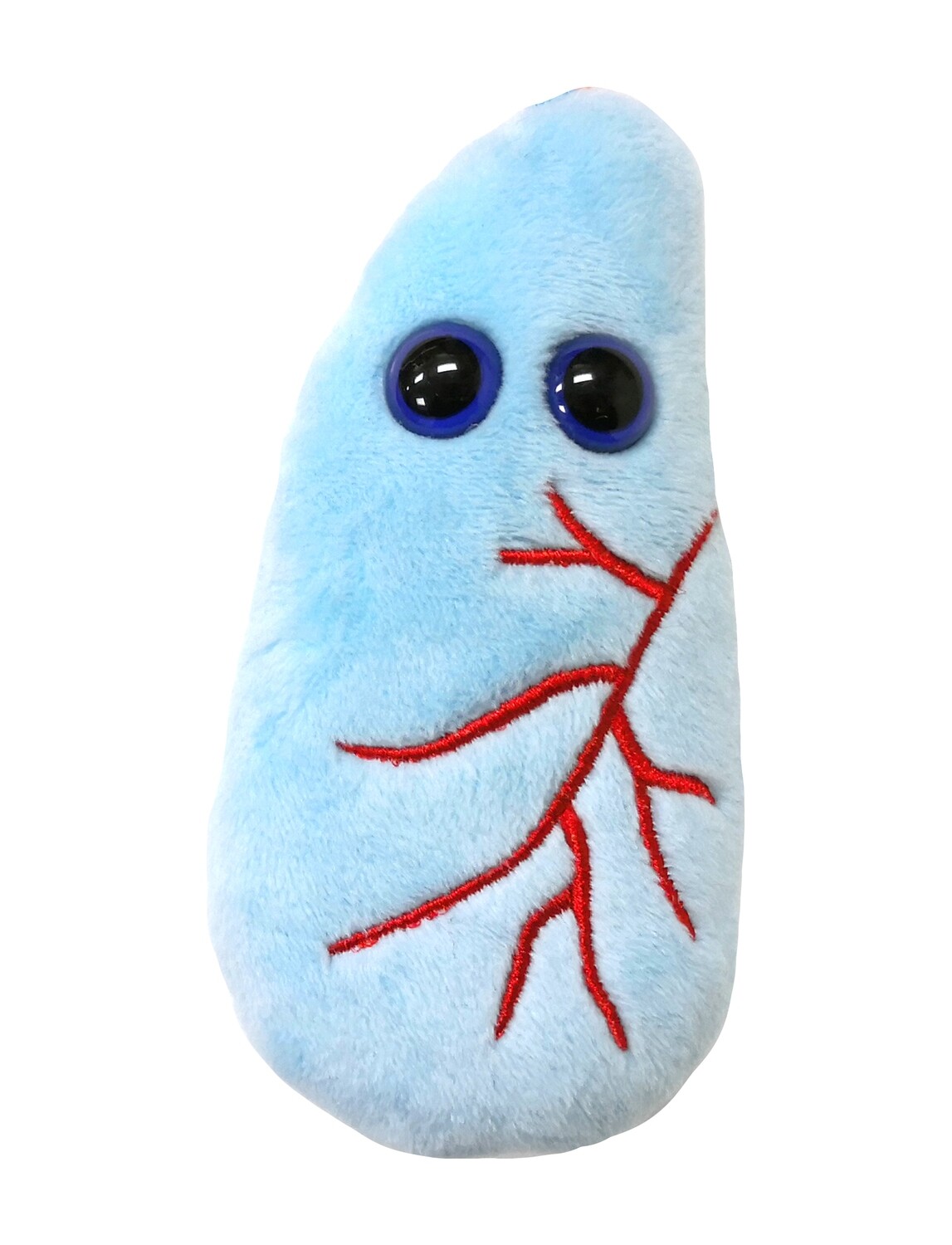
Methods of controlling microbial growth
Decades of research have developed the current understanding of microbial growth to establish the principles outlined above. Establishing common principles allows us to target broad groups of microbes, while unique requirements for growth allows us to target specific microbes. This knowledge enables the control of microbial growth that facilitates many of our interactions with microbes today.
Many methods of control seek to eliminate harmful microbes from foods or equipment. For example, high temperature is often used to kill microbes during cooking or through processes like pasteurization. In this way, potentially harmful microbes are broadly eliminated from the food product making it safe to consume and store. Similarly, chemicals in disinfectants can damage or kill microbes broadly on surfaces. Alcohols like ethanol and isopropanol damage the cell membranes. Without this protective structure, microbes cannot control what enters or exits the cell. Subsequently, microbes cannot retain important nutrients and water. Alternatively, hydrogen peroxide damages structures within the cell. As hydrogen peroxide decomposes, it forms molecules known as free radicals that damage proteins and DNA. Meanwhile, we also use soaps to physically remove microbes from surfaces. The chemical properties of soaps and physical force applied when wiping a surface dislodges the microbes.
When microbes cannot be completely eliminated from a material, such as food products that cannot be heated to high temperatures, measures can be taken to mitigate the growth of microbes. Recognizing how temperature impacts growth, supports the importance of refrigeration. As mentioned, cold temperatures slow the growth of microbes, so refrigeration can delay the growth of microbes in these food products. As described above, microbes can replicate as quickly as every 20 minutes leading to visible growth within only a few hours. At a lower temperature, the cells may divide only once every few hours and it will take multiple days to see visible growth.
Alternatively, when we want to take advantage of microbes, we try to optimize the conditions for their growth. Game 257: may 24 2019 the initials game show. This is why yeasted dough is left at a warm temperature to allow the yeast to grow rapidly. If the dough is refrigerated, it takes much longer to rise. Similarly, to use E. coli for insulin production, we provide bacteria with specific nutrients that facilitate growth.
Given the diversity of microbes in the world, it’s clear that there is still so much we don’t know about how they grow. Continuing to better understand microbial growth will help us live safely with the microbes in our community and make use of their unique capabilities.
Molly Sargen is a PhD student in the Biological and Biomedical Sciences Program at Harvard Medical School.
Nick Lue is a PhD student in the Chemical Biology Program at Harvard University.
Organism Chain
Cover image: “Bacteria sample inside petri dish for biotechnology study” by IRRI Images is licensed under CC BY-NC-SA 2.0
For More Information:
- Interested in understanding different types of microbes? Try this page.
- Learn more about the structure of bacterial cells here.
- For more details about cell division, see this page.
- For more details about mechanisms of microbial growth, read this article.
- To learn more about chemical disinfectants, check out this information from the CDC.
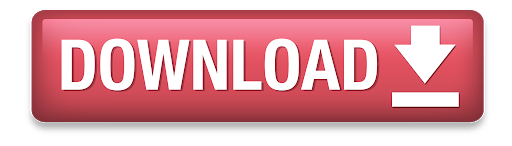